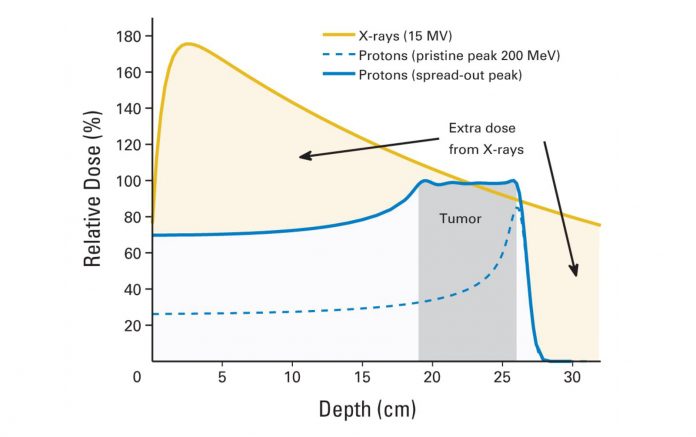
By Cynthia L. Kryder, MS, CCC-Sp
Posted: October 2017
For patients who present with inoperable, locally advanced lung cancer, photon-based chemoradiation remains the standard of care. Despite advanced radiation-delivery techniques, such as multi-leaf collimators, intensity-modulated radiotherapy (IMRT), and imageguided radiotherapy (IGRT), radiation oncologists continue to explore ways to extend the ALARA principle, that is, the desire to deliver tumoricidal radiation doses to intended targets while minimizing the radiation doses to adjacent healthy tissues. This has led radiation oncologists to investigate the potential of proton beam radiation therapy. In patients with non-small cell lung cancer (NSCLC), proton-beam therapy may enable safe dose escalation while sparing chest organs at risk and simultaneously maintaining adequate target coverage. In so doing, the collateral damage of standard radical thoracic radiotherapy can, theoretically, be mitigated.
Photons Versus Protons
Although the therapeutic index of modern, highly conformal photon radiotherapy has increased, the physics of photons make it impossible to avoid the exit dose downstream from the target, which is a physical limitation of the photon beam. In comparison, protons travel through tissue quickly and stop abruptly when reaching tissues at a very specific depth. Unlike photons, which deposit their radiation doses close to their entrance into the body, protons deposit most of their energy at the end of their paths, in a phenomenon known as the Bragg peak, the point at which the majority of energy deposition occurs. Before the Bragg peak, the deposited dose is about 30% of the Bragg peak maximum dose. Thereafter, the deposited dose falls to practically zero, yielding a nearly nonexistent exit dose. The integral dose with proton therapy is approximately 60% lower than any photon-beam technique.1 Thus, proton therapy delivers radiation to tumors and areas in very close proximity, decreasing integral radiation dose to normal tissues and theoretically avoiding collateral damage.
Despite these potential advantages, a fundamental issue with protons is the ability to stop the proton at the tumor. As any external beam travels through the body toward its target, it passes through tissues of different densities. Protonbeam therapy is much more sensitive to tissue density than photon therapy. Likewise, at greater depths the lateral margins of the proton beam become less sharp due to considerable scattering.2 Any change in tissue composition, such as organ motion, lung expansion, or alteration in bone position from one treatment to the next, can affect target coverage and dose to surrounding structures. To account for tissue heterogeneity and to reduce the potential for tumor underdosing, radiation oncologists often add a margin of uncertainty, meaning that the beam is designed to overshoot the target to guarantee good coverage.3 This could, however, negate the tissue-sparing advantage of proton-beam therapy and/or dilute its therapeutic effects.
Another difference between photon beam therapy and proton-beam therapy is the expense. Proton-beam therapy is an expensive technology. Including a cyclotron, multistory gantries, and several treatment rooms, the average cost for a proton facility ranges between US$140 million and US$200 million.
Assessing the Clinical Advantage of Proton-Beam Therapy
Given its lower integral dose and steeper dose gradient, proton therapy is an appealing therapeutic option. However, dosimetry advantages alone will not be enough to convince payors and patients to adopt this costly technology. Proton beam therapy must demonstrate a measurable clinical advantage when compared with standard photon therapy.
Clinical trials are underway to do just that. Zhongxing Liao, MD, of the Department of Radiation Oncology at the University of Texas MD Anderson Cancer Center, is the principal investigator of a multi-center, prospective, randomized phase III trial that will compare overall survival after photon versus proton chemoradiotherapy in patients with unresectable locally advanced NSCLC.4 This randomized trial will compare the overall survival (OS) in patients with stage II-IIIB NSCLC after image-guided, motion-managed photon radiotherapy (Arm 1) or after image-guided, motion-managed proton radiotherapy (Arm 2), both given with concurrent platinum-based chemotherapy. A total of 560 patients are expected to be enrolled. The primary endpoint is OS; secondary endpoints include 2-year progression-free survival, adverse events, quality of life, cost-effectiveness, and changes in pulmonary function.
A second ongoing trial seeks to determine whether the dose of radiation to the tumor, but not the surrounding healthy tissue, could be increased by using IMRT or intensity-modulated proton beam therapy (IMPT).5 In phase I of the study, investigators will identify the maximum tolerated dose (MTD) of IMPT and IMRT. In phase II, researchers will compare the efficacy of IMPT and IMRT when both treatments are combined with standard chemotherapy. The primary outcome measure is MTD; the secondary outcome measure is progression-free survival.
Future Outlook
The ability of proton-beam therapy to precisely target tumors and spare underlying tissues from radiation exposure in patients with a variety of cancers has already been demonstrated. Exactly if and how proton-beam therapy fits into the treatment of patients with lung cancer remains to be determined. Harnessing the power of proton-beam therapy in the treatment of NSCLC may be challenging given that protons must be delivered to the lungs, which are targets in motion that are surrounded by tissues of different densities. Future studies will need to assess not only side effects and outcomes, but they will also need to provide data to support the development of dose algorithms and motion-management techniques.
Given the capital investment and operating costs associated with protonbeam therapy, examining the economic advantages and liabilities of this new technology is necessary. Clear data about its cost effectiveness based on different clinical and treatment scenarios will enable providers, payors, and patients to make informed decisions about treatment. ✦
Expert Comment
The photon versus proton conundrum continues in the latter part of 2017, and it now must evolve in the context of promising new data with immune enabling drugs such as checkpoint inhibitors. Personally, I believe it is unlikely that further dose escalation to the target area will result in significant benefits in local control and overall survival from a radiobiologic perspective despite potential advantages in dose deposition by proton therapy, so newer directions are needed. From a cost perspective, is a 140-200 million monetary outlay for protons the way to get us to the promised land? Or will molecular and immunological discoveries offer the best avenue for success? Perhaps radiation, whether through protons or photons, will be the match rather than the flame for immune enabling drugs; therefore, dose escalation may be less important. Building on the theme of potential clinical advantages between photon or proton intensity modulated therapy, the question is whether less integral dose scatter within normal tissue with the use of protons will result in less chronic immunosuppression and thus potentiate checkpoint inhibition over photon irradiation. This is an amazing opportunity to study the changes in lymphocyte:neutrophil ratios during and after treatment. The bar has jumped with the anticipated results of the PACIFIC trial in locally advanced NSCLC, and we must jump with it. —David Raben, MD
References
1. Mitin T, Zietman A. Promises and pitfalls of heavyparticle therapy. J Clin Oncol. 2014;32:2855-2863.
2. Goitein M. Magical protons? Int J Oncol Biol Phys. 2008;70:654-656.
3. Paganetti H. Range uncertainties in proton therapy and the role of Monte Carlo simulations. Phys Med Biol. 2012;57:R99–R117.
4. ClinicalTrials.gov [website]. Comparing photon therapy to proton therapy to treat patients with lung cancer. Last updated June 10, 2016. https:// clinicaltrials.gov/ct2/show/NCT01993810. Accessed July 24, 2017.
5. ClinicalTrials.gov [website]. Intensity-modulated scanning beam proton therapy (IMPT) with simultaneous integrated boost (SIB). Last updated July 22, 2016. https://clinicaltrials.gov/ct2/show/NCT01629498. Accessed July 24, 2017.